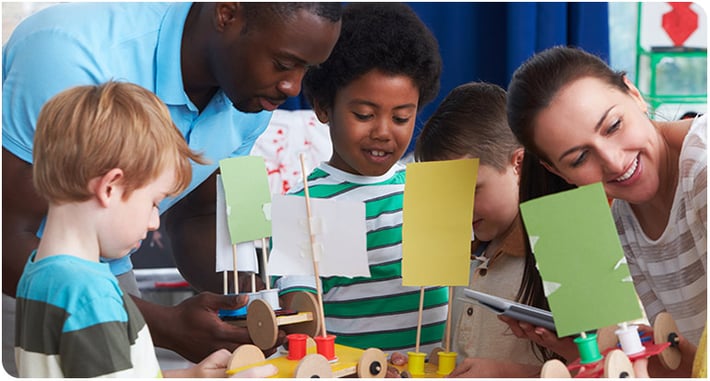
Updated on October 26th, 2023
An NGSS curriculum translates the Next Generation Science Standards into a classroom experience where students can be scientists and engineers. It’s what helps students gain experience performing science investigations and making connections on an everyday basis in order to reach mastery. Over time, they can generalize those skills in a variety of situations.
In order for this kind of learning to happen, it takes a science curriculum designed for NGSS.
NGSS Aligned Science Resources and Curriculums
The truth is that you can access resources from the National Science Teachers Association, educational companies, and other similar resources to find an almost infinite number of resources that claim to be aligned to NGSS.
But there’s a catch: If the resources are not, in fact, designed to support the standards, they fall short of the mark.
Many of these Next Generation Science Standards resources do not encourage higher-order thinking or build science and engineering skills. As a result, they fail to develop student mastery – the ability to face any question or problem and understand how to go about designing an experiment or prototype that will help them work toward an answer or solution.
Mastering NGSS means learning in an environment where students are challenged to figure out phenomena and form a personal connection to it through student-centered, hands-on instructional methods. Science standards based on DCIs, SEPs, and CCCs require learners to have the chance to achieve mastery of skills, content, and thinking in the context of phenomena. Lessons that provide hands-on learning experiences, but don’t give students the chance to plan and perform their own experiments, own the thinking, and then reflect on their own data, are not promoting deep mastery.
In contrast, genuinely NGSS aligned science curricula promote authentic critical thinking by requiring and challenging students' own ideas. The heart of a meaningfully aligned NGSS task is the challenge of thinking to develop an understanding of a concept, one’s own ideas, and personal connection to it. The task should require students to link concepts to real world examples that impact them by challenging them to define a path to evidence and design their own hands-on experiments to discover the answers.
Students engaged in well designed NGSS lessons are developing an identity as scientists and engineers in the classroom, and learning skills they will use in their future careers. Students who are only learning about what scientists and engineers do by reading about it, watching videos showcasing it, following canned tasks, recalling facts, or sitting behind screens disconnected from their peers are not building the deepest personal connections possible with the subject matter and the skills that are needed to perform true mastery.
And that skill of mastery is what NGSS requires.
When students are challenged to be scientists and engineers, they are actually developing the creative, evaluative, and analytical thinking skills that are useful for any college or career choice.
These higher-order thinking skills open a world of opportunity to any student. But to achieve that, classrooms need to be equipped with Next Generation Science Standards resources that are truly designed for—not merely aligned to—the standards.
When classrooms merely implement an NGSS aligned science curriculum, rather than one that is designed for NGSS, students can miss out on a much more meaningful learning experience.
On the other hand, a science curriculum that is truly designed for the standards will help students develop their critical thinking skills, meet the performance expectations of NGSS, and step into the shoes of scientists and engineers.
Often curriculum companies add an “aligned for NGSS” label to their product and list standards that they cover. However, for teachers and districts evaluating curriculum, it can be difficult to know how effectively the resources and NGSS sample lessons meet the goals.
That’s where we can help. Here are 3 key features all curricula truly designed for NGSS share.
Feature 1. NGSS Designed Curriculum Lessons Involve Real-World Context, or Anchor Phenomena
Every NGSS-designed lesson needs to involve real-world phenomena, what NGSS calls anchor phenomena, to drive student engagement and exploration.
Phenomena are the context in which scientists and engineers develop new knowledge. They are complex, real-world situations that can be explored in the classroom through an investigation that students or student teams have planned. Phenomena are a way of encountering a thread of often much more complex ideas.
Anchor phenomena can lead to both questions and problems. In either case, they should motivate students to investigate their ideas about the phenomena so they can either answer the question or solve the problem.
The anchor phenomena lead to questions and problems, which, in turn, lead to student dissatisfaction about what they know to be true about the phenomena. In other words, the aspects of the phenomena that students are trying to figure out lead to curiosity.
In the context of science, the question gives rise to a scientific investigation in which students plan to answer that question, carry out a hands-on experiment to observe the phenomenon for themselves, and then form an evidence-based conclusion based on the data they collect. That's the scientific process. It’s an experience, a thread of the anchor phenomenon that a student or student team can encounter hands-on.
In the case of engineering, an anchor phenomenon may give rise to a problem that students want to solve, in which students create a prototype that solves the problem. They may use available materials to design their prototype, test it, and then form an evidence-based conclusion with the data they collect. That’s the engineering process. It’s an experience with investigative phenomena that will yield data that students can use to reflect on whether or not their prototyped solution actually solves the problem.
Whether it's in the context of a question or a problem, what happens in the scientific process or the engineering process is investigative phenomena, where students are observing for themselves the results of their ideas in real time. They can use their data in an evidence-based conclusion, which is a critical part of the learning process. And then use their data and observations to reflect on what they now know or understand about the anchor phenomena.
An NGSS designed science curriculum takes into account the role of the student’s data and observations.
Example of an Anchor Phenomenon
An example of an anchor phenomenon might be flooding in the streets of New York City. There are many reasons that flooding might occur, but one possible scientific question related to this is, “How does water percolate through different earth materials?”
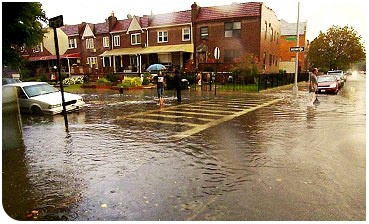
We can use a scientific process to investigate different earth materials and how water passes through them to come to an evidence-based conclusion about the impact on flooding of the materials that are found in the streets of New York City.
That same anchor phenomena can be used within an engineering context. Students might say, “The problem is that the streets of New York City are flooding. How can we fix that?”
Then students might come up with ideas about how the flooding could be solved. For example, they may have learned about tidal barriers, so they might design some prototypes, test them, gather the data, form a conclusion based on that data, and then reflect on whether tidal barriers would be a solution to the problem they have identified.
Dynamic Learning
When you can put anchor phenomena to work in the classroom using real-world contexts that enable students to reform their understanding of the world, the result is dynamic learning. Then students become scientists and engineers and develop higher order thinking skills. Dynamic learning is a hallmark that separates NGSS designed science curriculum from the merely aligned NGSS science curriculum.
That’s why it’s important to think critically about the Next Generation Science Standards resources you are using in the classroom.
Many classrooms use a K-W-L (What I Know, What I Want to Know, and What I Learned) model to guide student learning. However, a curriculum that is designed to engage students with phenomena so they can develop new knowledge often takes a “Why-How-What” model.
Using the “Why-How-What” Model to Integrate Real-World Phenomena
This “Why-How-What” (W-H-W) model is a modification of the standard K-W-L chart, which asks students to list out what they already know, what they want to learn in the lesson, and what they learned at the end.
Instead of trying to start the student experience with what they know, start with why. In the case of KnowAtom, we use nonfiction text, visuals, and sometimes even videos as the beginnings of anchor phenomena.
The purpose is to establish a reason to care. We want to connect with what students believe, giving them a reason to care about, say, flooding the streets of New York City.
Several questions we can put to students are: Why do we care about flooding in New York City? Why does flooding occur? Why do some areas flood more than others?
Once students have a reason to care, the next type of question to ask is how. For example, how does flooding occur? How do the properties of the ground surface affect the amount of flooding that occurs? In the case of engineering, how can the effects of flooding be reduced?
The final question to ask is what. What are the elements in the anchor phenomenon that we can explore? What are the problems or questions that we see with one or more aspects of the phenomenon?
This is where students become dissatisfied with the evidence they have to explain or argue what they believe to be true about the anchor phenomenon and where they examine their belief to find out what's really behind it. What evidence do they have for believing it?
If they say it's because the street is concrete, how do they know that it's because the street is concrete? What we're doing is moving from the anchor phenomenon toward investigative phenomena.
What kind of investigation will lead to evidence that might solve our dissatisfaction with what we know about how water and concrete interact, for example, or what it means when sea levels rise or how tidal barriers work?
This leads to the next feature that distinguishes an NGSS designed science curriculum from an NGSS aligned science curriculum.
Feature 2: NGSS Designed Lessons Help Students Learn How to Plan Their Own Investigations.
The expectation of these new standards is that students engage as scientists and engineers as the means of developing their understanding. The emphasis is on students’ developing and using the content as scientists and engineers, engaging in risk-taking and problem solving, and explaining through investigative phenomena.
In other words, what’s important is that students can perform independently. What is their skill set? That’s the important question. Your skill set as a teacher needs to help support your students in their productive struggle through that planning process. This helps them internalize the skills and develop them as a habit when they encounter something that requires investigation or does not meet their framework of understanding.
This feature is closely related to Feature 1 because part of the challenge here is to engage students in a complex real-world situation that causes them to be dissatisfied in some way with the evidence they have to argue about what they know, what they can explain, or with the fact that this phenomenon even exists.
The key part of Feature 2 is that a properly designed NGSS curriculum puts the student in the position of being dissatisfied with the evidence they have to explain or argue what they believe to be true about the anchor phenomena. This leads to student questions or problems, and the curriculum supports students as they learn how to plan their own investigations based on those questions or problems.
The scientific question or engineering problem stems from the WHW and leads to the investigative phenomenon, which students will approach either through a scientific or engineering process. From there, they gather evidence to form their conclusion, which then adds to their beliefs and gives them more reason to be invested the next time they ask, “Why?”
The problem or question becomes the entire platform for a student-led investigation so that the students can form conclusions based on their data.
Upon gathering evidence and forming a conclusion based on their experiments, students then relate their own experiences to that initial anchor phenomenon. They now have the necessary real-world, interactive, investigative experience needed to revise their framework of understanding and gain real skills and meaning from the lesson or unit. An NGSS designed science curriculum facilitates this naturally; whereas, a merely NGSS aligned science curriculum does not always deliver the interactive experience necessary for meaningful connections.
The science and engineering practices are key to this next generation shift because they establish the skills that students need to develop in order to approach problems and questions with an eye toward solutions and answers. When students take on the role of scientist and engineer, they use the 8 NGSS practices to develop and use the content as they authentically answer questions and solve problems.
Yet content is not enough anymore; it is the formation of skills, and the ability to develop and use content, that is so vital to the classroom experience today. This type of inquiry can happen anywhere and at any age —with the right Next Generation Science Standards resources.
Feature 3: The NGSS Standards are Taught in Groups
The final feature of an NGSS-designed science curriculum - as opposed to only an NGSS-aligned science curriculum - is that the standards are taught in groups, not in isolation. When we teach standards in groups and relate them to one another through crosscutting concepts, students can begin to master three-dimensional learning.
An NGSS aligned curriculum promotes real world science learning, as students master concepts while practicing how to engage like inquisitive scientists and engineers. A key component to their success is understanding that these career fields are dynamic. Scientists are continuously searching for new discoveries and engineers are constantly experimenting to uncover new solutions. The job of a scientist is to answer questions through data based experimentation while engineers solve problems by developing and testing prototypes that later become evidence based solutions.
Providing students with three-dimensional learning experiences means developing a classroom culture where DCIs, SEPs, and CCCs are experienced and manipulated to figure out concepts. Working actively in groups, students achieve mastery by sparking each other's thinking and feeding off new and different ideas as they take the lead in developing a personally meaningful understanding of their own agency and the gain the benefits of working with others.
Cooperative learning allows teachers to release responsibility to their students, as they work together to ask questions, choose what problems they want to learn more about, and find their own answers through hands-on scientific inquiry. Teachers in an authentic NGSS classroom are skillful questioners, engaging students in reflection as they formatively assess and develop their own understanding of each student's performance
The Fourth Dimension of NGSS: The Nature of Science and Engineering
In addition to the well-known three dimensions of the Next Generation Science Standards, there is a somewhat lesser-known dimension called the nature of science and engineering. This dimension defines science and engineering and explains how they are connected in the STEM cycle.
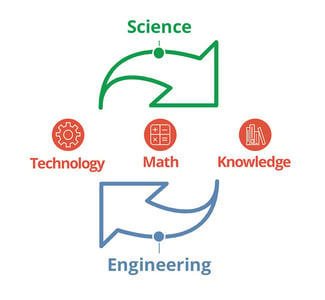
The nature of science and engineering is that they form a connected cycle, in which science adds new knowledge that engineers use to solve problems, and engineers, by solving problems, enable scientists to fine-tune their queries and generate newer and more detailed knowledge. Technology, math, and knowledge facilitate the cycle.
NGSS-designed curriculum needs to integrate this dimension as well so that students understand the context in which they are developing the three dimensions of content, systems thinking, and skills.
For example, the 4th dimension of NGSS clearly defines science as knowledge from experimentation. When your students are talking about science, they should be talking about knowledge from experimentation. If they're talking about being a scientist, they should be talking about answering questions through experimentation – planning and using experiments to gather data and grow their own knowledge.
Similarly, in an engineering context, the process is about taking that scientific knowledge and using it to solve identified problems. An engineer, quite simply, identifies and solves problems. Taking the knowledge of science and identifying and solving problems before coming to evidence-based conclusions about prototypes is exactly how engineers go about trying to solve a problem, and we can replicate this closely with our students. They create a prototype solution. They test it. That's how they get data to inform themselves about that potential solution. If it actually solves the problem, it can then become technology.
Technology enables scientists to ask new and deeper questions through the scientific process, and so the cycle feeds itself. In fact, engineers improve on technology that other engineers create through the course of time and ideas—as well as through the development of new knowledge discovered by scientists.
The reason you see math at the center of this cycle is that it’s a tool for communication and for quantifying, analyzing and evaluating observations. It's a key part of both science and engineering.
In a classroom that understands and leverages the true nature of science and engineering, students are scientists and engineers on an everyday basis. They are mastering those processes as tools to be able to answer questions and solve problems.
This type of education relies on an integrated approach with a dynamic framework that pulls the ideas of earth science, life science, physical science, and engineering/ applications of technology not only through the year, but from one grade level to the next.
Discover Next Generation Science Standards Resources
Districts that want to successfully implement the Next Generation Science Standards will need to make sure that any curriculum they choose is actually designed for the standards. That’s why it’s important to be able to identify if NGSS aligned science curriculum is merely superficially aligned to the standards or not.
NGSS curriculum that is designed for the NGSS standards has the following features:
- 100% phenomena-driven, with the phenomena as the real-world motivation and basis for students to figure out how or why something happens
- A three-dimensional learning experience where students learn to plan their own investigations so that they develop the skills to expand on what they know across different disciplinary core ideas and crosscutting concepts
- Fully integrated lessons and units that scaffold skills and concepts throughout the year and from one grade level to the next
The nature of science and engineering refers to the disposition a student should take when developing solutions and discovering new information about the world around them. The disposition of those engaged in the disciplines of science and engineering professionally are as active, evidence based investigators who appeal to different methods of accessing evidence as part of their work. An NGSS curriculum should engage students in the nature of science and engineering by structuring a learning environment with tasks that require the authentic disposition of a scientist or engineer.
If you would like more information, please reach out. We would be happy to have a conversation with you about how we might help to meet the needs of your students, your classroom, or your district. You can also download NGSS sample lessons or browse all Know Atom's next generation science standards resources.